
Lunar Science Priorities
Within NASA’s Science Mission Directorate (SMD), the Exploration Science and Strategy Office (ESSIO) leads NASA’s efforts to achieve our Moon to Mars (M2M) science objectives.
These objectives are derived from Astrophysics, Planetary, Heliophysics, Earth, and Biological and Physical Sciences’ Decadal Surveys. For example, M2M’s Lunar and Planetary Science objectives (LPS-1, LPS-2, and LPS-3) are closely aligned with the top three lunar priorities within the 2023-2032 Origins, Worlds, and Life (OWL) Planetary Decadal. OWL’s science questions were accompanied by over 60 Moon-related strategic research topics. NASA’s Endurance-A and Lunar Geophysical Network (LGN) are recommended mission that significantly address LPS-1 and LPS-2, while LPS-3 is being addressed through Commercial Lunar Payload Services (CLPS) and Artemis human missions.
Below, we’ve outlined some of the high-priority lunar science objectives from the Planetary Decadal survey and M2M that NASA’s Endurance-A and LGN missions will tackle, furthering our understanding of the Moon and paving the way for future exploration.

Understand the correlation between the Moon’s history and the evolution of life on Earth
We can learn about the history of life on Earth by studying the Moon. It’s possible the origins of life on Earth were influenced by events in other parts of the solar system. We may be able to establish lunar geological or compositional changes that coincided with major evolutionary events on Earth.
Current science shows that Earth most likely received the bulk of its carbon, nitrogen, and other volatile elements essential for life from the planetary collision that created the Moon more than 4.4 billion years ago. Endurance samples from the largely unexplored South Pole Aitken (SPA) Basin will provide invaluable data insights to scientists and big historians that could change our understanding of the origins our world and life.

Understand the Earth and Moon formation
Analysis of samples from NASA’s Apollo missions have shown that the Earth and Moon share nearly the same isotopic composition. Scientists have used compositional data from lunar samples, along with impact modeling, to hypothesize that the Earth and Moon are the product of a massive collision between a proto-Earth and a Mars-sized planetary body called Theia. However, without active seismometers on the lunar surface, it’s difficult to establish anything definitive about the Moon’s bulk composition and interior structure.
The lunar interior holds the keys to unlocking many more secrets about the formation of Earth, the Moon, and the entire solar system. For example, if the size of the Moon’s core turns out to be much larger than thought, the origin story of our planets would change drastically!
We have some data from seismometers deployed by the Apollo astronauts, but a clearer picture of the Moon’s interior makeup can be ascertained by adding more seismometers in disparate surface locations. LGN would place at least four nodes in wide-ranging regions around the Moon.

Establish the age difference between lunar crustal and mantle rocks
The Moon has been around for over 4.5 billion years, so it’s safe to say it has gone through some changes over time. Based on data from the Apollo return samples, scientists have inferred that the Moon was originally mostly molten, then cooled down and solidified over time. However, return surface samples from SPA Basin via Endurance will provide a brand-new data set from a previously unexplored region of the Moon.
SPA Basin is one of the largest and oldest impact features in the solar system, and impactors (comets, meteors, etc.) have left it with a very thin crustal layer, potentially opening access to the lunar mantle and making it possible for Endurance to obtain samples of some of the solar system’s oldest geological materials. The chemical composition of Endurance lunar crustal and mantle samples combined with in-situ data from Endurance’s scientific payloads will provide scientists with a crucial piece of the puzzle in understanding the formation of the Moon, Earth, and even other planetary bodies.
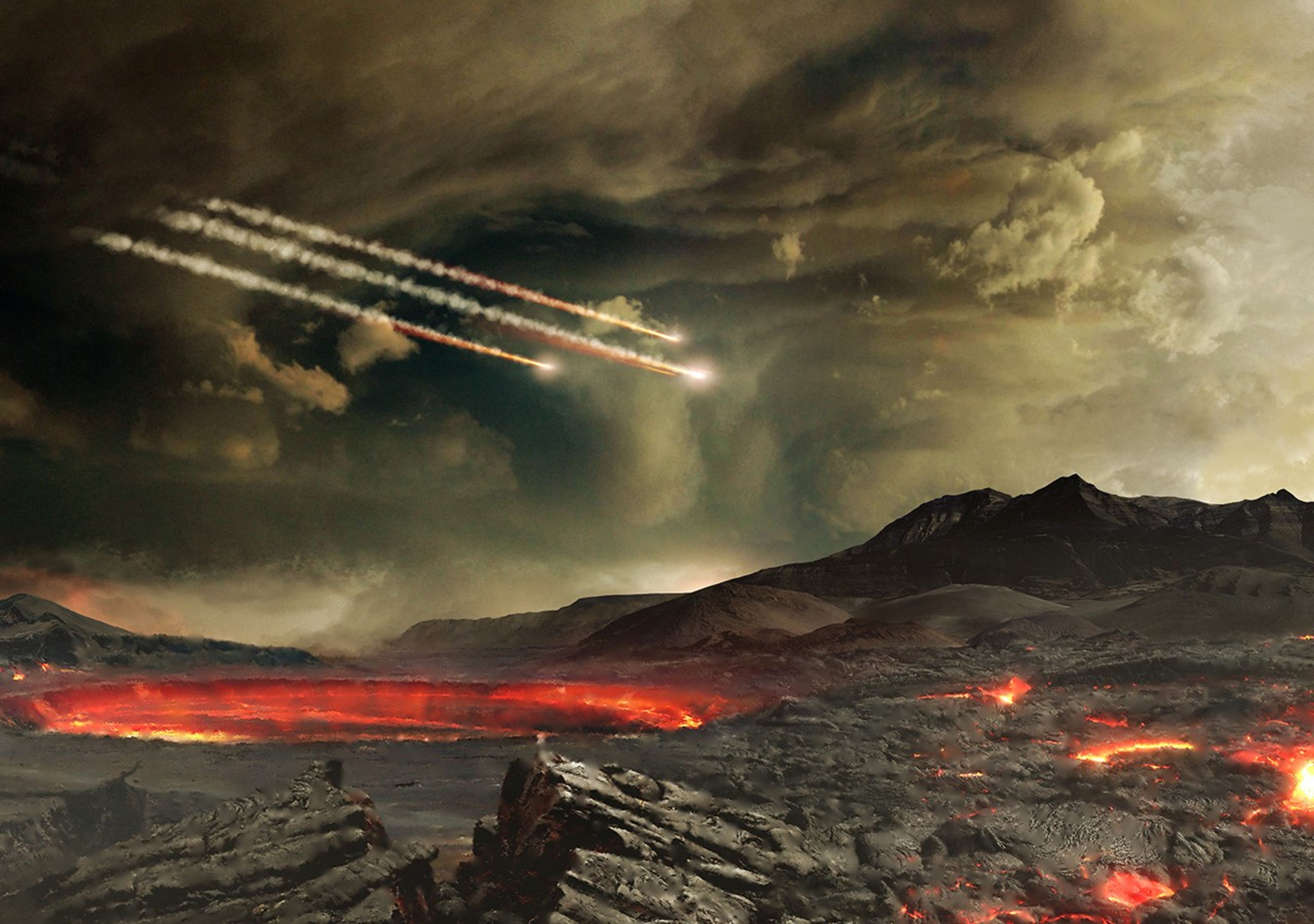
Study the history of Late Heavy Bombardment era
As the earliest-formed surface area of the Moon, the South Pole-Aitken Basin is a treasure trove of information that will provide new context to all lunar and planetary science. In this case, the SPA Basin may be able to tell us more about the theorized Late Heavy Bombardment that is thought to have occurred approximately 4 billion years ago.
The Late Heavy Bombardment era had a massive influence—it may have even caused planets to swap orbits 🤯. SPA Basin’s surface samples may hold answers about this time. With a better understanding of the Late Heavy Bombardment’s significance and timing, we can clarify the geological and geophysical history of all planetary bodies in the solar system.
We’ll establish much more accurate ages of key impact basins across the Moon by performing isotopic dating on surface samples returned by Endurance.
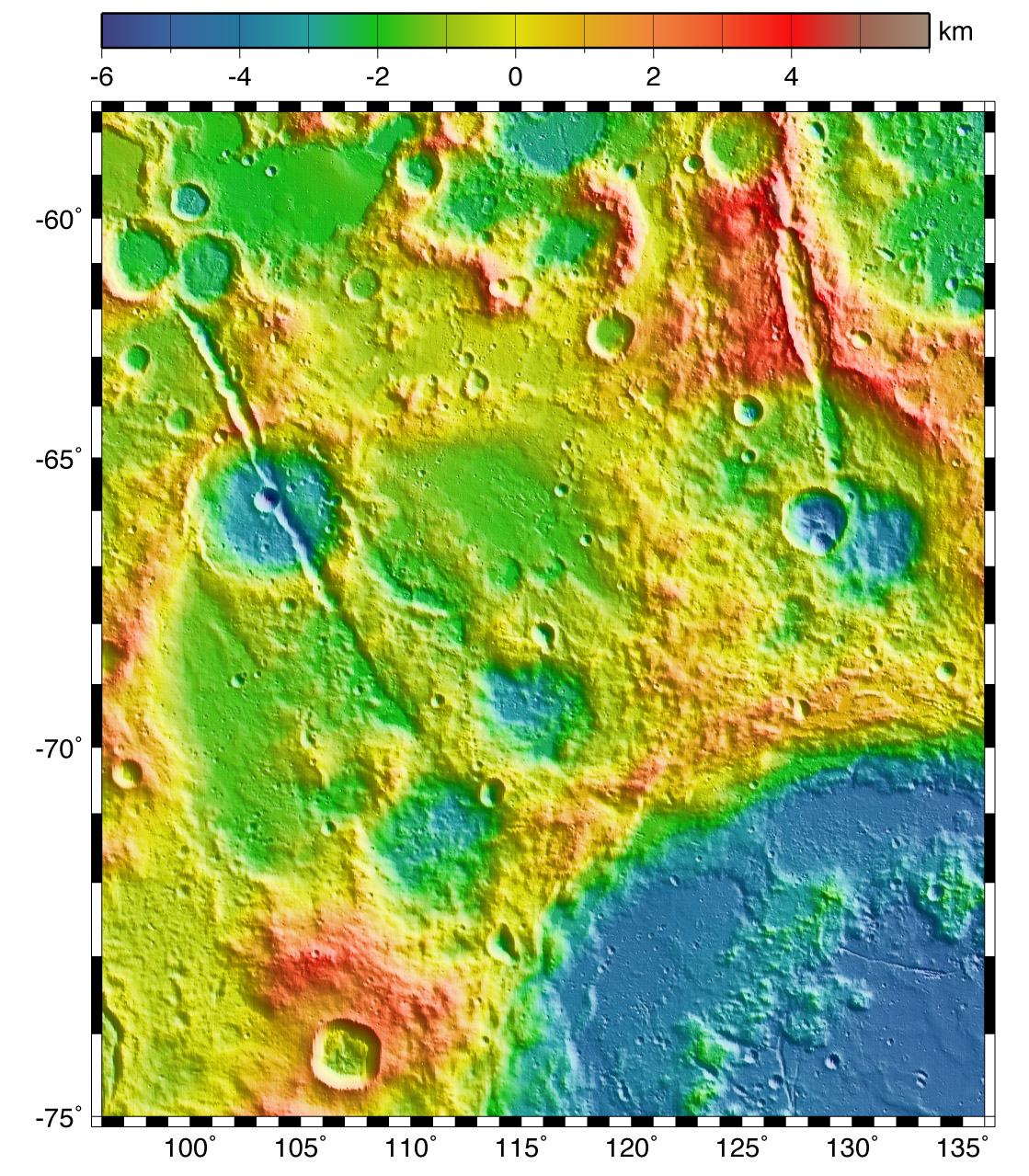
Better understand the Moon’s impactors
If you look closely at the Moon’s surface, you can tell it’s gotten its fair share of scars from impacts over billions of years. Studying these bombardment areas can tell us much about our planetary history.
Relative to the rest of the planets in our solar system, the Moon and Earth are so close to each other they can practically high five (and we like to think they would). So, if there were periods of time where the Moon was getting bombarded by impactors that contained specific materials, we can infer that Earth received similar materials as well.
Endurance would return surface samples from significant impact locations, where scientists will seek information about the materials these impactors were carrying. What was hitting the Moon, and when? How were materials distributed? Even more: was water one of the materials delivered? Were the building blocks of life imported to both the Moon and Earth, and Earth was simply better suited to foster that growth? Endurance surface samples may hold the keys to understanding the origins of life!

Establish a precise, absolute lunar impact chronology that can be applied to other worlds
All science is based on accurate standards from which to measure data. For instance, if a baker’s scale is zeroed incorrectly, every following measurement will be inaccurate, wasting a bunch of time and flour. Similarly, it’s crucial to establish a precision baseline chronological measurement for lunar impactors, as they inform the science community’s models of the origins of our solar system.
Endurance will enable us to measure the radiometric ages of surface samples extracted from terrains determined to be older than 3.9 billion years and comparing those with the radiometric ages for terrains younger than 3 billion years, we’ll be able to establish a more accurate resolution with which to measure the chronology of planetary events.
Additionally, obtaining a larger sample size from a greater variety of locations should enormously boost our data set from which lunar cratering history is modeled. Understanding the relationship between surface crater density and age will be a massive breakthrough in calibrating the lunar timeline. Any changes to lunar impactor timeline will shift our understanding of collision events throughout the solar system.

Study the composition and depth of South Pole Aitken Basin materials
The Moon’s composition is very similar to that of Earth, but they aren’t identical. SPA Basin samples will provide a unique and expanded context for scientists in establishing the origins of these materials, and therefore the origins of the Moon and Earth.
Samples collected by Endurance will also help explain why the crust is significantly thinner on the lunar near side than on the far side. SPA Basin’s relatively thin crust not only suggests that lower crust or upper mantle components were exposed or incorporated into impact melt, but also gives scientists easier access to these samples.

Calibrate the Solar System’s timeline
Based on the isotopic analysis of samples returned from the Apollo missions, scientists have hypothesized that between 4.1 and 3.8 billion years ago, the lunar surface was heavily bombarded by asteroids. Study of this so-called Late Heavy Bombardment (LHB) has essentially anchored the chronology that is used as a basis for understanding the absolute timing of events throughout the solar system. In a way, lunar chronology is the solar system’s timekeeper. This means understanding the timing of lunar impactor events has far-reaching implications on what we know about the rest of the solar system’s history.
The present-day lunar impact rate can give researchers clarity about lunar chronology. By using LGN seismometers coupled with lunar observations of impact flashes, fresh impact craters, and sample returns from Endurance, we will learn much more about the timeline of the solar system.

Establish the processes that shaped the Moon’s interior structure
Many planetary bodies, including Earth, were formed via volcanic and magmatic processes. And as we’ve mentioned, tides influence the formation of planetary crusts and interiors. But how did exogenic (exterior) and endogenic (interior) events affect the Moon’s makeup? How do those events relate to tidal influence? We want a better understanding of these relationships to illuminate the past, current, and future of the Moon, Earth, and the rest of the solar system.
Both LGN and Endurance will help us answer these questions. LGN will monitor seismic activity, electromagnetic sounding data, and heat flow around magma plumes, which will allow scientists to compare current heat production with past heat production and provide insight into the change in magmatic processes over time. Data provided by analysis of Endurance surface samples will tell us more about the volcanic processes that helped shape the Moon.

Better understand the Moon’s internal structure
We have a considerable understanding of the Earth’s internal composition because Earth is accessible to us. We’re confident we have a good idea of the Moon’s composition, but soon we’re going to know a lotmore—and there are teams working on doing the same for Mars. We want to understand how and why these bodies differ from one another.
NASA has limited data about the internal layering of our Moon based on returns from the clustered Apollo seismic network. That data is certainly helpful, but the geographic placement of the seismometers limits seismic insights. Geographically and temporally expanded seismometer data will provide much more accurate clarification of bulk composition.
LGN will provide crucial seismic and heat flow data needed to establish a clear and accurate picture of the Moon’s interior, which may even further our understanding of the interiors of other planetary bodies.

Establish tidal influence on crustal structures
Crusts, or the outermost layer of a planet, are paramount to understanding planetary bodies, and tidal influence is a major factor. In this case, establishing the tidal influence on the Moon’s crust will provide context and clarity for all crustal measurements, as well as clarifying if and how tidal activity deforms the Moon’s structure.
LGN data will illustrate a much more complete picture of the Moon’s interior, which should provide data that will allow scientists to better understand tidal influence throughout the solar system.
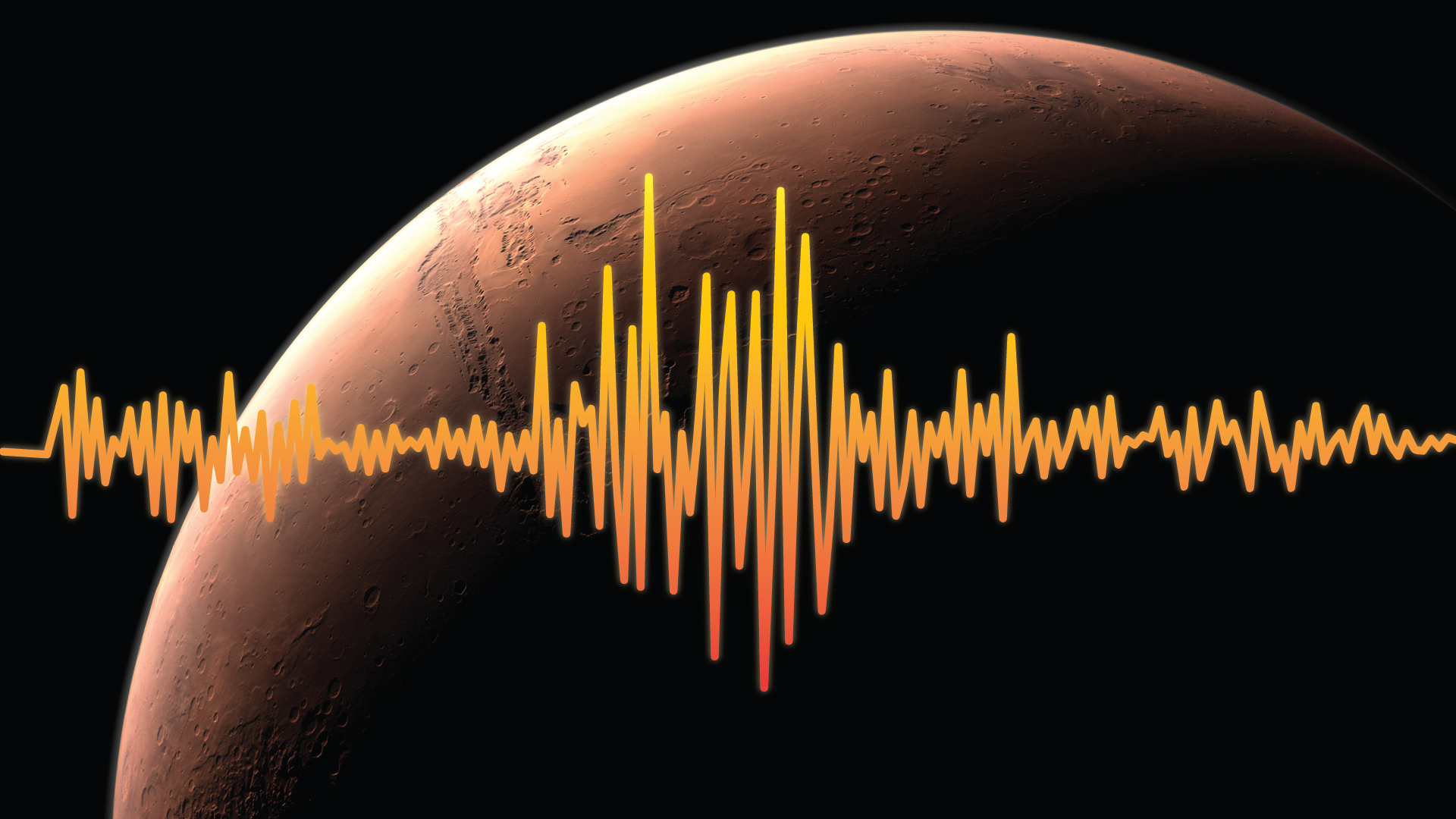
Understand the style and intensity of tectonic activity on other planetary bodies
Earth is considered the most tectonically active planetary body in our solar system. Our planet exists and evolves via a set of geological and scientific processes unique to Earth. But our understanding of the tectonism on most other planetary bodies is largely theoretical, based on mineralogical and geological studies using remotely sensed data.
However, there is a way to enhance the accuracy of these hypotheses. By gaining a seismic understanding of planetary bodies like the Moon and Mars, we’ll be able to radically sharpen our theories about the tectonism of planets elsewhere in the solar system. Even distant Pluto’s icy exterior won’t stop us from understanding its internal composition!
Just as InSight provided groundbreaking seismic data on Mars, LGN will do the same on the Moon—and hopefully all planetary bodies in our solar system.

Measure the history of the Moon’s magnetic field
Based on all currently available data, scientists presume the Moon doesn’t produce a magnetic field. But we also don’t think it’s always been this way.
The Moon’s theorized former magnetic field, generated by the liquid outer core until between two and four billion years ago, holds answers to questions about the formation of the Moon and Earth. Over time, that core cooled and solidified, and the Moon lost its magnetic field. There are geomagnetic anomalies in different locations around the Moon, something we’re very curious about. These spots might help unlock the magnetic (and therefore volcanic and magmatic) history of the Moon. How did the Moon lose heat over time?
LGN’s seismometers, magnetometers, and electromagnetic sounding devices will provide high-resolution measurements that will allow us to study the orientation of known rocks in the Moon’s interior, which should be able to tell us much more about the direction of the Moon’s long-lost magnetic field.