Overview
Earth's Moon is covered in craters. Humanity's fascination with these telltale remnants of impact events is entwined with our understanding of our companion satellite.
Lunar impact craters are the depressions left behind after an asteroid, meteoroid, or comet collides with the Moon. Though craters are visible on worlds throughout the solar system, lunar craters are special because we can see them close-up, and the relative lack of erosion and other surface processes on the Moon keep them well-preserved.
Craters fascinate us because they are a record of the process that built and shaped our entire solar system, the fingerprints of history pressed into the Moon’s surface.
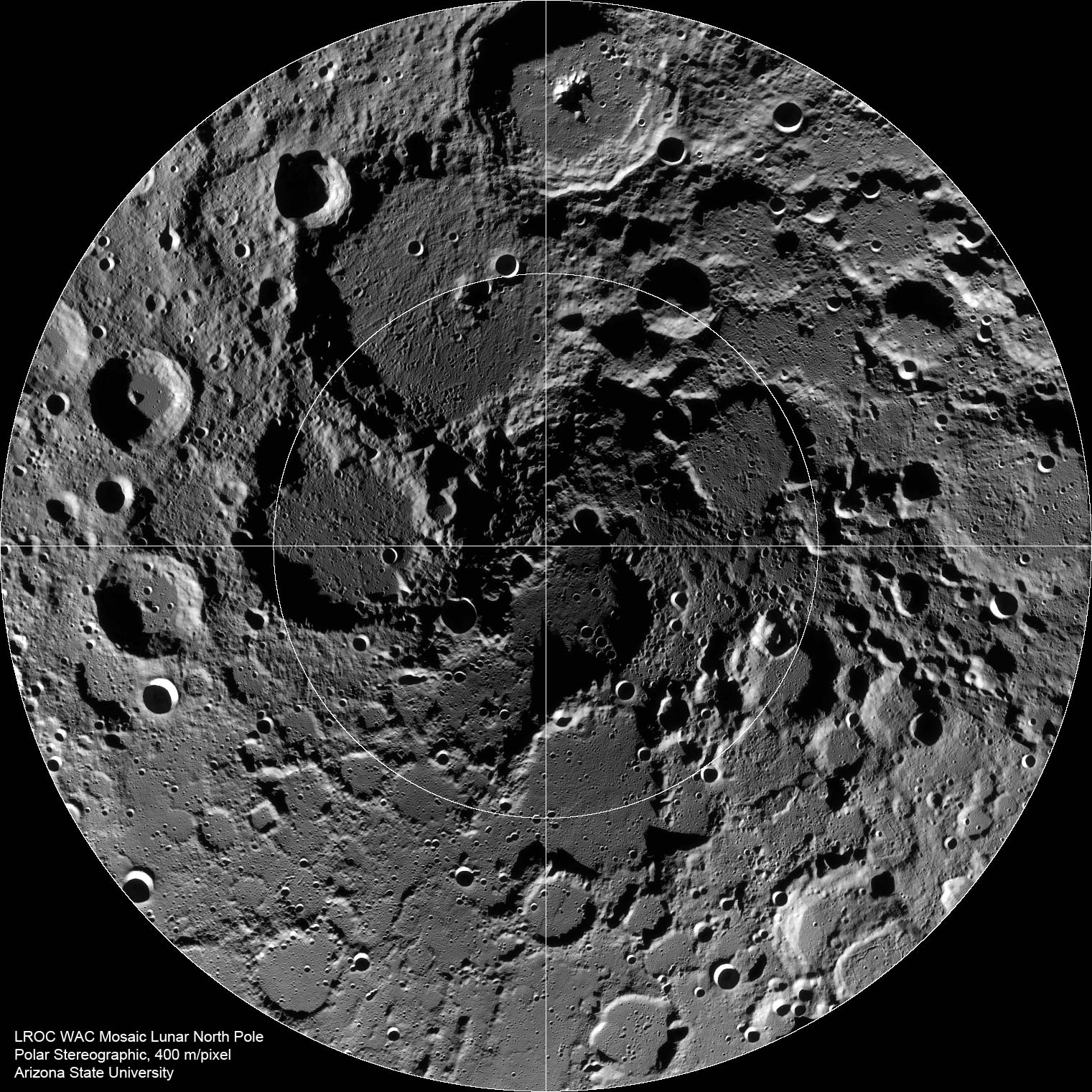
Solar systems form through collision. Tiny flecks of dust strike and stick to one another, gradually transforming through accumulation into pebbles, boulders, and planetoids, and then finally into planets. The bits and pieces left behind by this process are still here as the rocky and icy objects that populate areas in our solar system like the asteroid belt, Kuiper Belt and Oort Cloud.
Collisions continue even today, with solar system bodies periodically pummeled by these leftovers. The meteoroids, asteroids and comets left swirling about the Sun are occasionally drawn into a gravitational embrace with their bigger siblings. On Earth, erosion and plate tectonics slowly erase their imprints. But when we look at the sky, the Moon’s craters are visual proof of the messy process that led to our planet’s creation.


Types of Craters
Simple craters
...are typically what people would sketch out if asked to draw a crater. They look smooth and are shaped like bowls with round floors. Simple lunar craters tend to be on the small side, no more than around 6-9 miles (10-15 km) in diameter.
Complex craters
...are larger than simple craters and have features like central peaks, terraces and flat floors. On the Moon, complex craters are generally around 9 miles (15 km) or more in diameter. (On Earth, they form at a much smaller size because of our planet’s stronger gravity.)
Basins
...are enormous craters that are more than 186 miles (300 km) in diameter on the Moon. Lunar samples suggest that most of the major basins on the Moon formed around 3.9 billion years ago in a still-mysterious period called the Late Heavy Bombardment. Over time, many basins filled with magma, creating the dark, basaltic Moon "mare" that we can see with the unaided eye from Earth. There are more than 40 impact basins on the Moon, and they play a significant role in the Moon’s geology. The impacts that created the Moon’s basins were intense enough to not just melt rock but also to influence volcanic flows from the Moon’s mantle.


How Craters Form
A lunar crater forms when an asteroid, meteoroid, or comet, typically moving faster than the speed of sound, plunges into the Moon’s surface. The energy of the object ― determined by its size, density and speed ― as well as the type of surface it hits and the angle at which it hits, all factor into what kind of crater forms in this explosive impact.
Crater formation has two phases: excavation, when the initial hole in the ground forms, and modification, when the shockwaves and their aftermath affect the entire area around the impact, causing the ground to deform and collapse. The largest craters are relatively shallow compared to their diameter due to modification, while the smaller craters keep their original deeper bowl shapes.
When an impactor contacts the Moon’s surface, it compresses, pulverizes, and vaporizes the rock beneath it. That material then rebounds, and the energy of the rock decompressing sends shockwaves racing outward through the surface, melting some of the rock if the pressure becomes high enough. Material shoots upwards and outwards. We call this spray of pulverized rock “ejecta.”
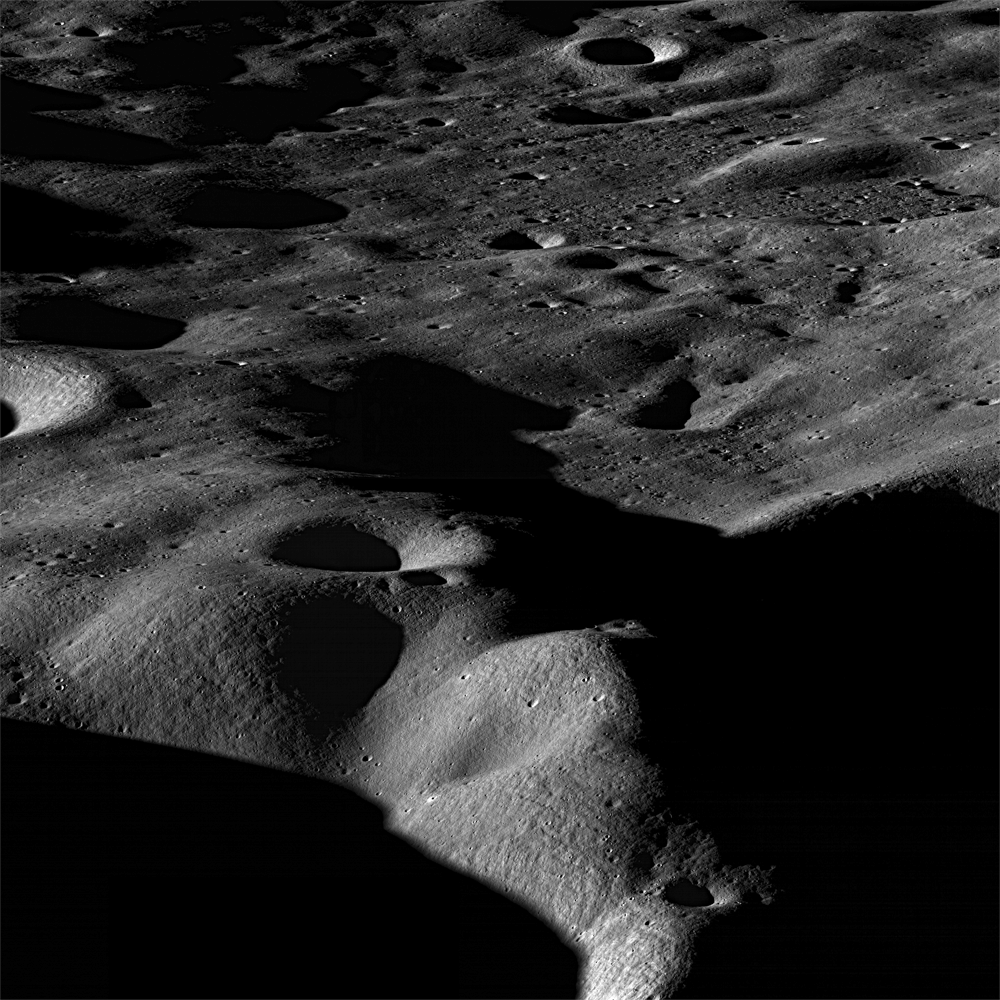

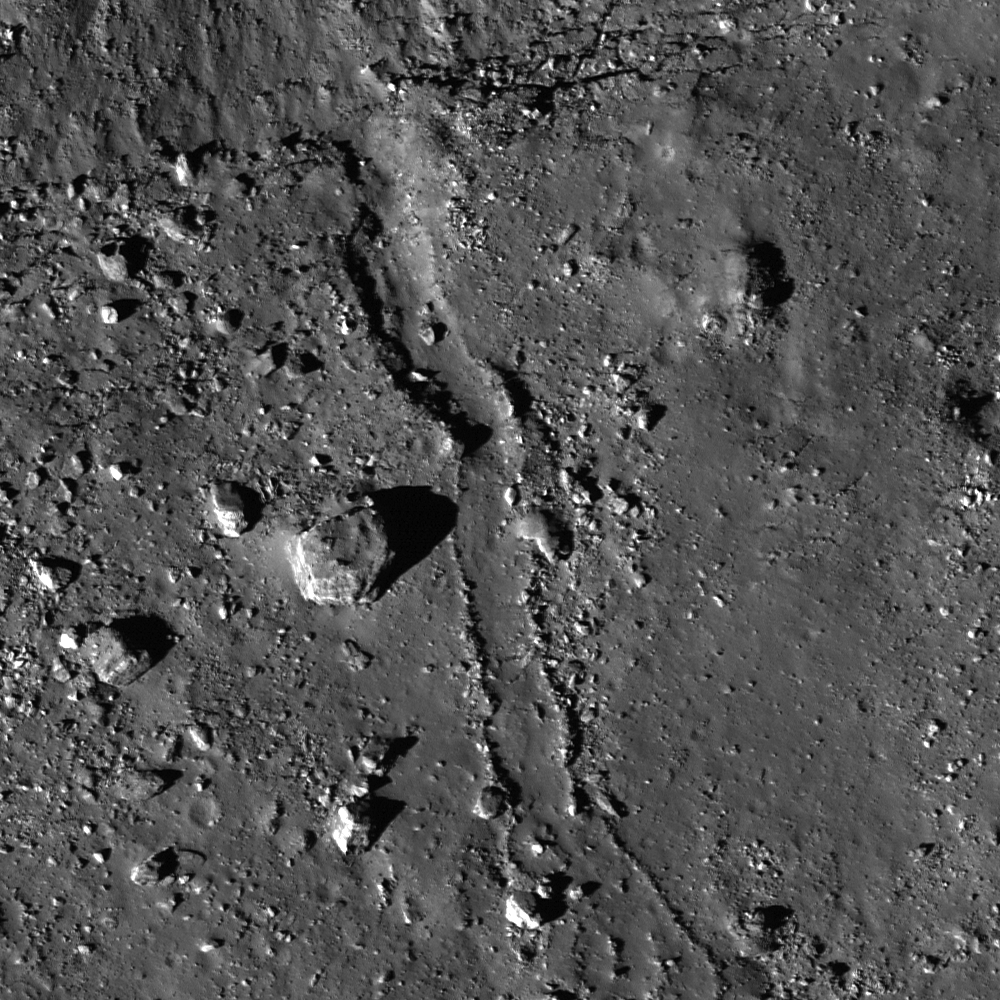
Some of the ejecta may be large enough to cause secondary craters when it crashes back to the surface ― these smaller craters will later be seen as chains or clusters of craters pointing back to the main crater. Most of the ejecta spreads out in the area around the crater, thicker and more uniform nearest the crater. Plumes of ejecta that settle back onto the surface form the bright rays seen radiating from many of the Moon's craters. And a portion of the ejecta may even reach orbit, as evidenced by lunar meteorites found on Earth.
When the energy of the impact is powerful enough, the rebounding material stops acting like a solid and behaves like a liquid. Like the rebound from a water droplet splashing into a puddle, the liquefied rock can form a peak in the center of the crater that rises and then collapses. As the rebound forms that central peak, material from the crater rim rushes in to fill the suddenly empty space. Large blocks of rock begin to fault and slump inward, expanding the diameter of the crater and creating the stepped features called terraces. If the impactor has even more energy, clusters or even rings of peaks can form as the crater’s central material rises and then gets pulled back by gravity.
The rock at the crater’s surface settles and cools into a layer of something called lunar breccia, a type of igneous rock made up of coarse, angular, shattered fragments of older rocks glued together by molten rock. Beneath that, the bedrock will have fractured, the cracks eventually tapering out as they plunge deep into the crust.

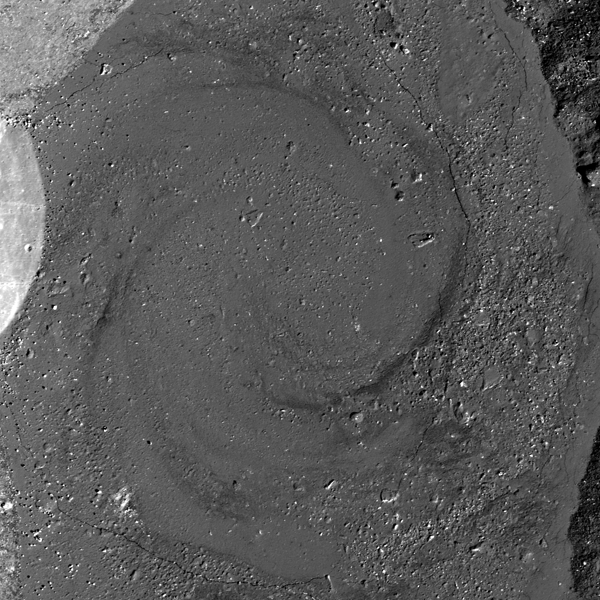

How Basins Form
Lunar basins are just giant craters, the aftermath of world-transforming impacts. The space rocks that created the Moon’s basins are arguably more responsible than any other factor for the appearance of the Moon we see in the sky ― and for much of its geology.
The “maria” that make up the faces and characters that people imagine in the Moon are giant pools of basalt, an igneous rock formed from the magma that welled up through cracks in the crust created after impactors carved basins into the lunar surface.
Basins are defined as being more than 186 miles (300 km) in diameter. They can be multiple times larger than the excavation of the initial crater, expanding as the territory around them is wracked and reshaped by impact-generated forces. The largest basin on the Moon, the South Pole-Aitken Basin, is thought to be four times larger than its initial excavation.
During basin formation, the initial impact and its rebound removes and melts so much material that the entire area becomes structurally unsound, causing faults to form in the surrounding land and the walls of the crater to collapse inward, drawing in material from a wider region. The initial impact crater is erased by the intensity of the aftermath.
Some basins are surrounded by multiple rings. The process of ring formation is still being studied, but scientists suspect that the rings are the result of a combination of faults and the rise and collapse of peaks that form during the impact.
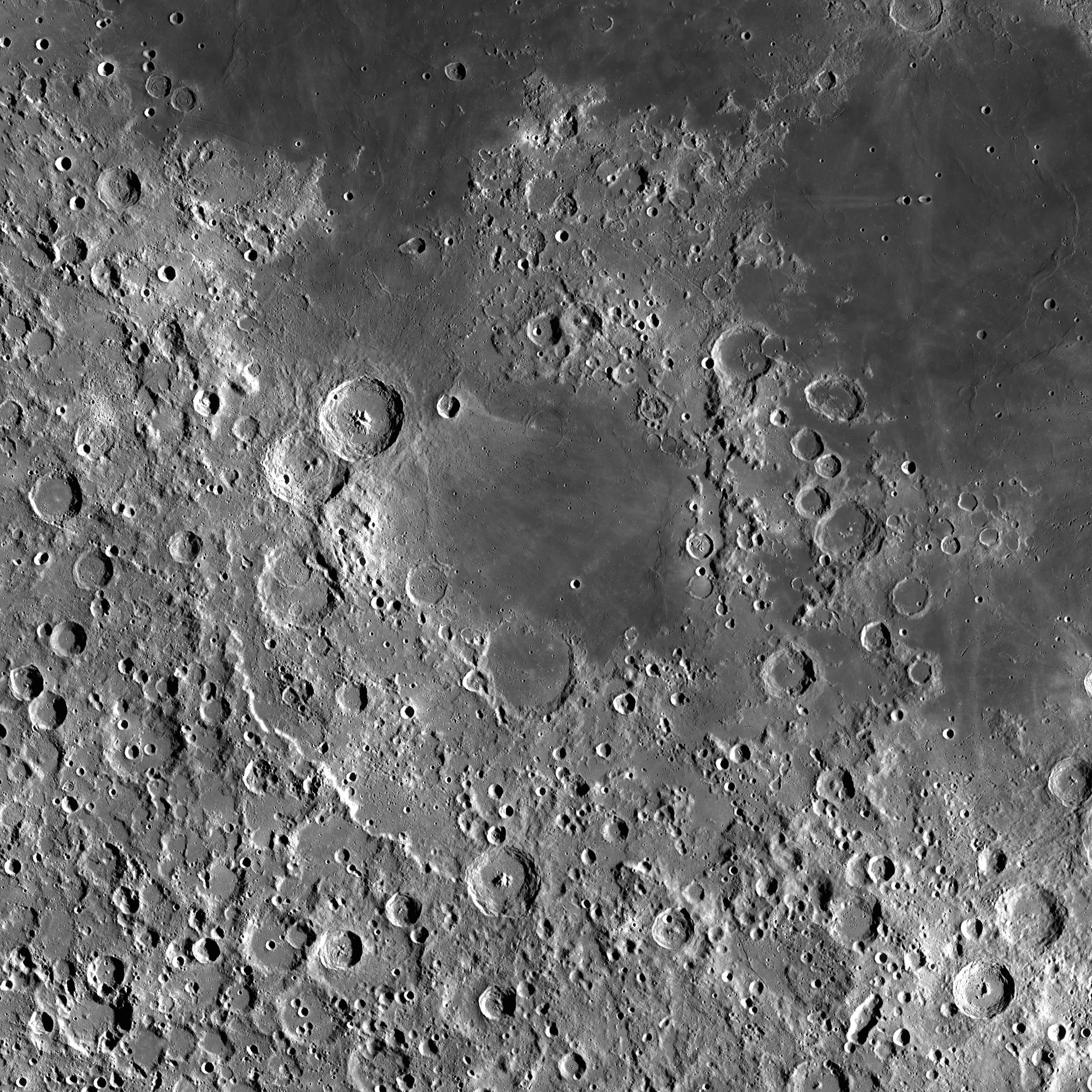
Another key feature of basins are the smooth floors left behind by pooled melted rock, or “impact melt.” When a typical crater forms, material that melted in the impact can be scattered and tossed out of the crater as ejecta. This impact melt can be visible at the surface, flowing down the slopes of the crater or draping features. It can also be found as tiny fragments in breccias.
When a basin forms, some melted material also scatters, but the depth of the melting is so extensive ― kilometers to tens of kilometers deep ― that a substantial amount remains in the basin. In the case of the giant South Pole-Aitken Basin, the solidified impact melt sheet is thought to be 31 miles (50 km) thick.
It can take millions of years for such deep pools of melted rock to cool, and during that time any new impactors will strike a thin crust over a thick layer of molten rock, leaving no craters behind and making the area appear young to observers. As these melt sheets cool and crystalize they contract, creating depressions in the centers of the basins. These depressions can remain visible even after they are covered by basalt deposits from later volcanic flows.
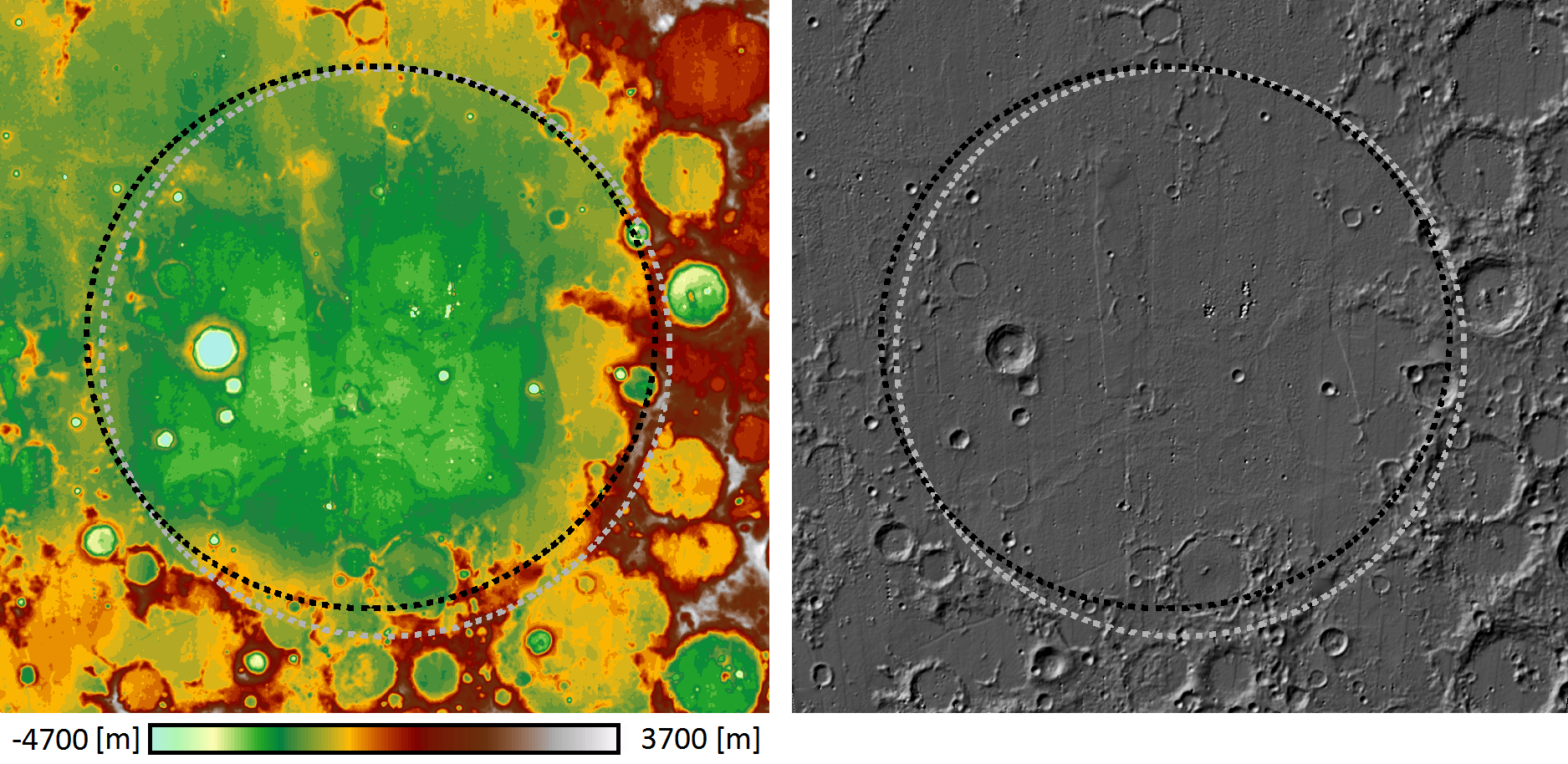
The color-coded map on the left helps show Nubium due to a slight depression easily seen in the topographic map. Parts of a rim structure can be identified in the southeast, suggesting a basin diameter of about 419 miles (675 km) (black dashed line), which is consistent with previous estimates of 428 miles (690 km) (gray dashed line). The model to the right accentuates the smooth floor of Nubium.
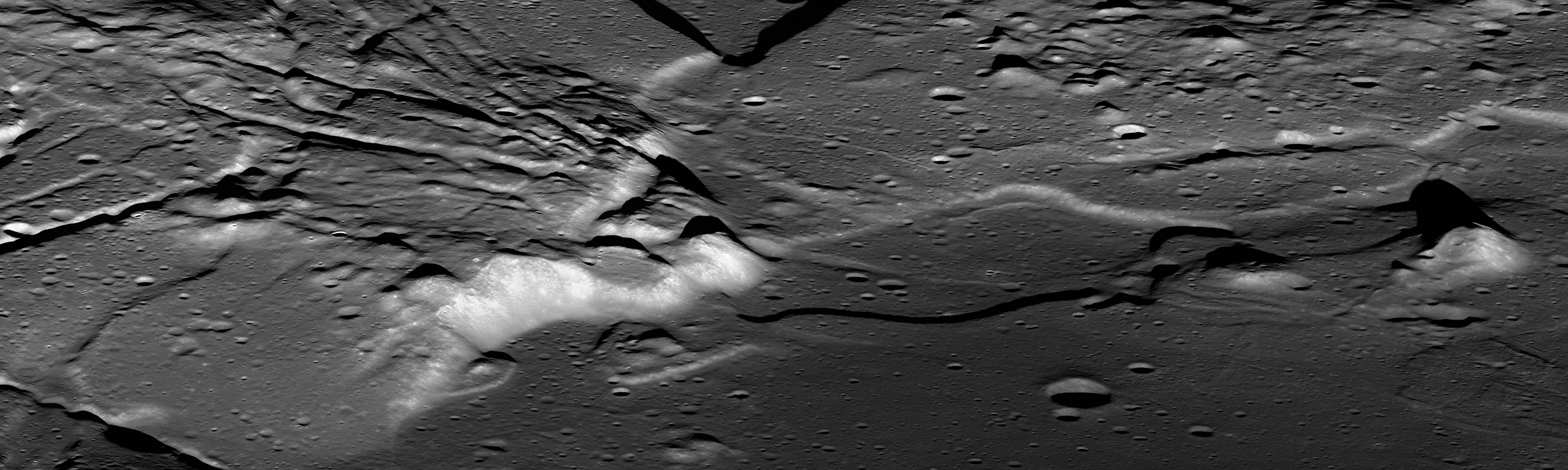
Writer: Tracy Vogel; Science Advisors: Daniel P. Moriarty (University of Maryland at College Park), Natalie M. Curran (NASA's Goddard Space Flight Center)
Explore Further
Expand your knowledge about lunar craters.
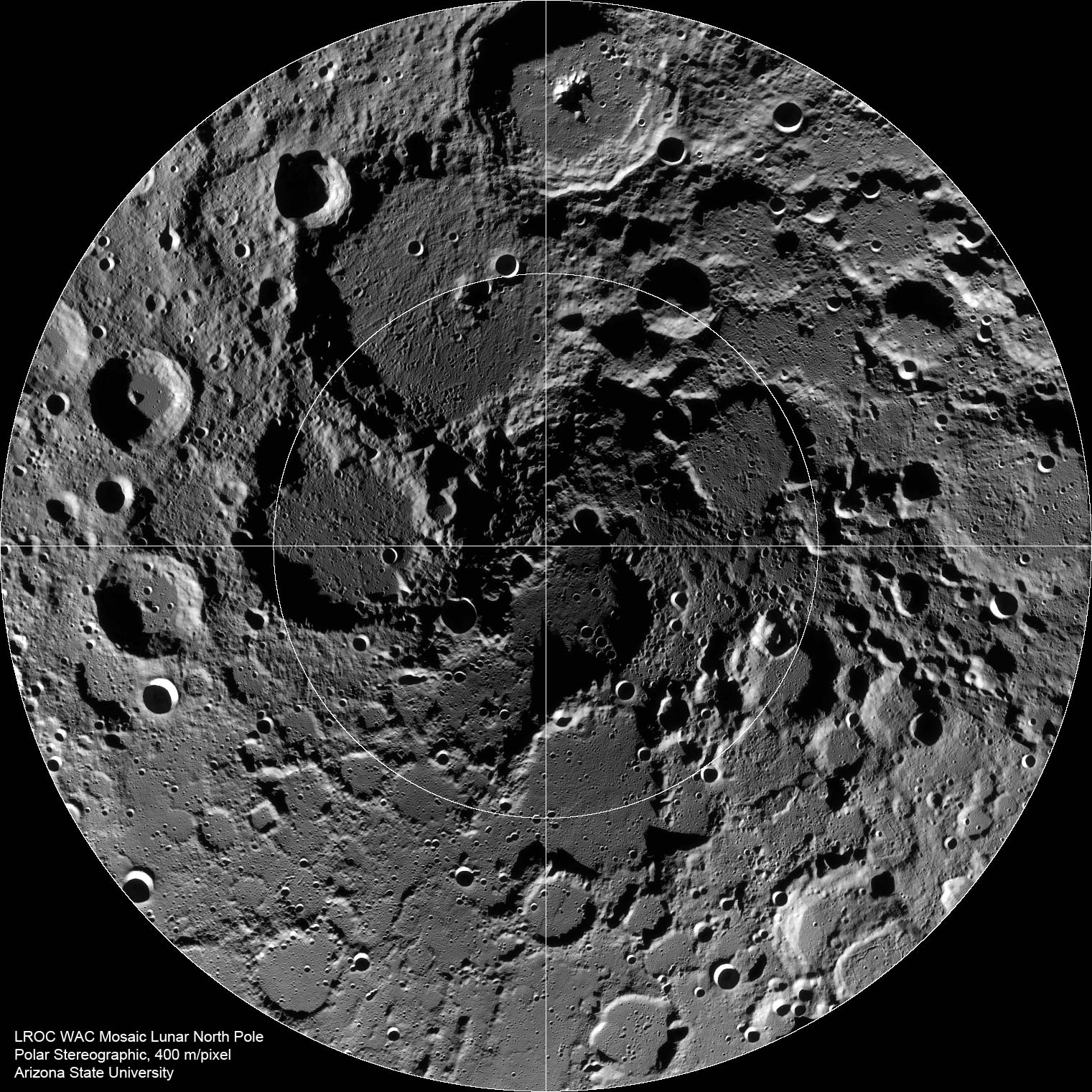

What is the Late Heavy Bombardment?
Lunar craters give scientists a peek into our solar system’s asteroid-pummeled past.


The Explosive History of Orientale Basin
The Moon’s Orientale basin demonstrates the violence of a tremendous lunar impact.