Using All Our Senses in Space
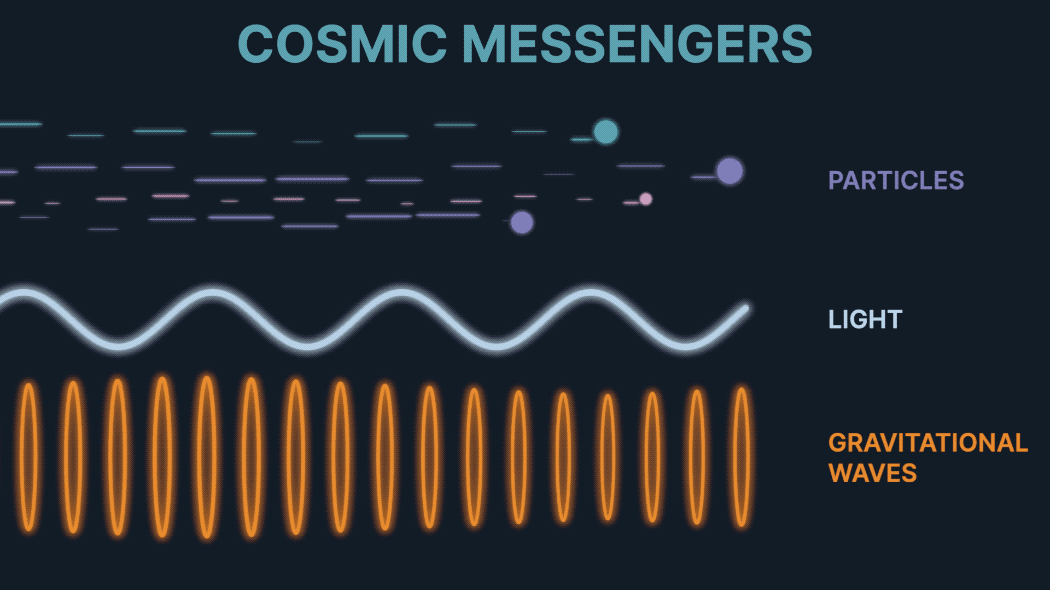
We experience the world through different senses: sight, touch, taste, hearing, and smell. Similarly, astronomers now study the universe using different messengers: light, particles, and space-time ripples called gravitational waves. They can learn much more about cosmic objects and events by combining information from multiple messengers than by using just one. Scientists call this approach multimessenger astronomy.
Light
Scientists picture light as streams of photons, particles representing the smallest possible packets of light energy. Light behaves both as if it’s made up of particles and as if it’s made up of waves. While we usually talk about light in terms of waves, it’s really a bit of both.
Different types of light combine into a collection called the electromagnetic spectrum. Scientists define these different regions of the spectrum by the energy, wavelength, and frequency of their light.

Radio waves have the lowest energies, lowest frequencies, and longest wavelengths of the electromagnetic spectrum. On Earth, we use radio waves to transmit data when we use cell phones or wireless internet. The universe is also full of radio-emitting objects like pulsars, nebulae, and some types of galaxies.
Microwaves cook popcorn in a few minutes. Astronomers use this light to learn about the structure of galaxies, including our own. NASA’s Cosmic Background Explorer (COBE) used this wavelength to map the cosmic microwave background, taking a sort of baby picture of the universe.
Infrared light is often used to detect heat. It’s what you see when you wear night-vision goggles. In space, astronomers use it to map the dust between stars and observe newborn stars. NASA’s James Webb Space Telescope employs infrared light to study some of the universe’s oldest stars.
Visible light is what our eyes detect. It’s emitted by fireflies, lightbulbs, and stars. Scientists use it to study all kinds of cosmic objects. NASA’s Transiting Exoplanet Survey Satellite, for example, uses visible light to find exoplanets, or worlds beyond our solar system.
Ultraviolet (UV) light carries more energy than visible light. Most of it is blocked by Earth’s atmosphere, but some UV rays from the Sun get through it to give us tans and sunburns. NASA’s Hubble Space Telescope uses UV light to study everything from auroras on Jupiter to star formation in other galaxies.
X-rays are so penetrating that dentists use them to see into your teeth and airport security uses them to see through bags. Astronomers study X-rays emitted by neutron stars, galaxies, and cosmic explosions with missions like NASA’s Chandra X-ray Observatory.
Gamma rays are the highest-energy form of light. They’re used to sterilize food and medical equipment and to treat some forms of cancer. Astrophysicists use them to investigate some of the most powerful events in the cosmos – explosions of massive stars and collisions of neutron stars and black holes. NASA’s Fermi Gamma-ray Space Telescope has been collecting gamma rays for over a decade and has discovered many exciting new phenomena, including two huge gamma-ray bubbles extending thousands of light-years above and below the center of our home galaxy.
Particles
Many cosmic objects and events also eject high-speed particles. In some cases, particles allow scientists to peer into places that cannot be seen with light. For example, some particles can escape extremely dense environments where even light might struggle to get out, like inside dying stars. Cosmic particles come in two classes –neutrinos and cosmic rays.
Neutrinos
Neutrinos are fundamental particles – they can’t be broken down into smaller parts. They’re produced by radioactive decay and nuclear reactions, including those that occur in nuclear reactors on Earth, in the cores of stars, in supernova explosions, and when black holes shred stars. Neutrinos outnumber all the atoms in the universe, travel at nearly the speed of light, and rarely interact with other matter. These characteristics make neutrinos interesting, but also very hard to study.
The National Science Foundation’s IceCube Neutrino Observatory in Antarctica uses a series of instruments buried in a cubic kilometer of Antarctic ice to detect neutrino signals. Even with that volume, IceCube only sees one neutrino every six minutes. That’s one neutrino for every 500 trillion trillion that pass through the observatory in the same amount of time.
NASA’s Goddard Space Flight Center
Cosmic rays
Cosmic rays are charged, high-energy particles that move through space at near-light speed. Every second, every square meter of Earth’s atmosphere is pelted by large quantities of cosmic rays. These particles are usually hydrogen nuclei, or protons. But scientists have detected examples of other subatomic particles like neutrons, electrons, and even antimatter. NASA’s Super Trans-Iron Galactic Element Recorder (SuperTIGER), a balloon-borne instrument, detected cosmic rays composed of the nuclei of heavier atoms, like cobalt or barium.
Cosmic rays are boosted to high speeds in cosmic events and environments that release a lot of energy. One example is the expanding debris of exploded stars called supernova shock waves.
Since cosmic rays are charged – positively charged protons or nuclei, negatively charged electrons – their paths through space can be deflected by the magnetic fields of stars and other objects.
Gravitational Waves
Gravitational waves are ripples in space-time, the fabric of the universe, that travel at the speed of light. Gravitational waves are created when objects speed up, slow down, or change direction. And just like light, they come in different wavelengths and frequencies. All accelerating masses generate gravitational waves, including pencils, people, and planets. But we can currently detect only those produced by superdense stellar remnants – black holes and neutron stars. Even the strongest waves detectable at Earth distort our planet’s shape by only about 1% the size of an atom.

Albert Einstein predicted the existence of gravitational waves in 1916 in his general theory of relativity. Scientists gained confidence in their existence by watching the orbits of some binary systems but didn’t directly observe them until 2015 when the National Science Foundation’s Laser Interferometer Gravitational Wave Observatory (LIGO) detected ripples from the collision and merger of two stellar-mass black holes. The upcoming European Space Agency-led Laser Interferometer Space Antenna (LISA) is designed to detect gravitational waves from pairs of supermassive black holes in merging galaxies.
Scientists are also using pulsar timing arrays – specific sets of millisecond pulsars, rapidly rotating cores of stars that exploded as supernovae – to hunt for some kinds of gravitational waves. Millisecond pulsars sweep beams of radiation, from radio to gamma rays, past our line of sight, appearing to pulse with incredible regularity – like cosmic clocks. As long gravitational waves pass between one of these pulsars and Earth, they delay or advance the light arrival time by billionths of a second. By looking for a specific pattern of pulse variations among pulsars of an array, scientists expect they can reveal gravitational waves rolling past them.