4 min read
Who among us doesn’t covertly read tabloid headlines when we pass them by? But if you’re really looking for a dramatic story, you might want to redirect your attention from Hollywood’s stars to the real thing. From birth to death, these burning spheres of gas experience some of the most extreme conditions our cosmos has to offer.

All stars are born in clouds of dust and gas like the Pillars of Creation in the Eagle Nebula pictured below. In these stellar nurseries, clumps of gas form, pulling in more and more mass as time passes. As they grow, these clumps start to spin and heat up. Once they get heavy and hot enough (like, 27 million degrees Fahrenheit or 15 million degrees Celsius), nuclear fusion starts in their cores. This process occurs when protons, the nuclei of hydrogen atoms, squish together to form helium nuclei. This releases a lot of energy, which heats the star and pushes against the force of its gravity. A star is born.

From then on, stars’ life cycles depend on how much mass they have. Scientists typically divide them into two broad categories: low-mass and high-mass stars. (Technically, there’s an intermediate-mass category, but we’ll stick with these two to keep it straightforward!)
Low-mass stars

A low-mass star has a mass eight times the Sun’s or less and can burn steadily for billions of years. As it reaches the end of its life, its core runs out of hydrogen to convert into helium. Because the energy produced by fusion is the only force fighting gravity’s tendency to pull matter together, the core starts to collapse. But squeezing the core also increases its temperature and pressure, so much so that its helium starts to fuse into carbon, which also releases energy. The core rebounds a little, but the star’s atmosphere expands a lot, eventually turning into a red giant star and destroying any nearby planets. (Don’t worry, though, this is several billion years away for our Sun!)

Red giants become unstable and begin pulsating, periodically inflating and ejecting some of their atmospheres. Eventually, all of the star’s outer layers blow away, creating an expanding cloud of dust and gas misleadingly called a planetary nebula. (There are no planets involved.)

All that’s left of the star is its core, now called a white dwarf, a roughly Earth-sized stellar cinder that gradually cools over billions of years. If you could scoop up a teaspoon of its material, it would weigh more than a pickup truck.

High-mass stars
A high-mass star has a mass eight times the Sun’s or more and may only live for millions of years. (Rigel, a blue supergiant in the constellation Orion, pictured below, is 18 times the Sun’s mass.)


What’s left behind depends on the star’s initial mass. Remember, a high-mass star is anything with a mass more than eight times the Sun’s — which is a huge range! A star on the lower end of this spectrum leaves behind a city-size, superdense neutron star. (Some of these weird objects can spin faster than blender blades and have powerful magnetic fields. A teaspoon of their material would weigh as much as a mountain.)

At even higher masses, the star’s core turns into a black hole, one of the most bizarre cosmic objects out there. Black holes have such strong gravity that light can’t escape them. If you tried to get a teaspoon of material to weigh, you wouldn’t get it back once it crossed the event horizon — unless it could travel faster than the speed of light, and we don’t know of anything that can! (We’re a long way from visiting a black hole, but if you ever find yourself near one, there are some important safety considerations you should keep in mind.)
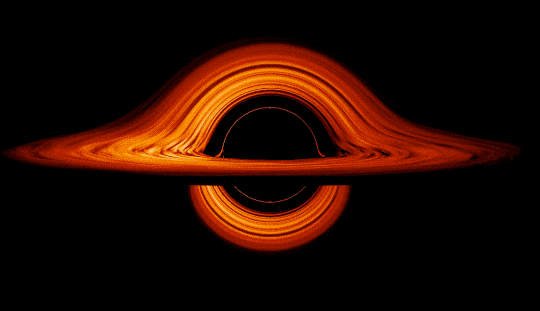
The explosion also leaves behind a cloud of debris called a supernova remnant. These and planetary nebulae from low-mass stars are the sources of many of the elements we find on Earth. Their dust and gas will one day become a part of other stars, starting the whole process over again.
That’s a very brief summary of the lives, times, and deaths of stars. (Remember, there’s that whole intermediate-mass category we glossed over!)