PROJECT:
Advanced Magnetic Microcalorimeter development
SNAPSHOT:
A new class of X-ray detector with unprecedented energy resolution and array size could help transform our understanding of the cosmos through unparalleled vision into the otherwise invisible universe.
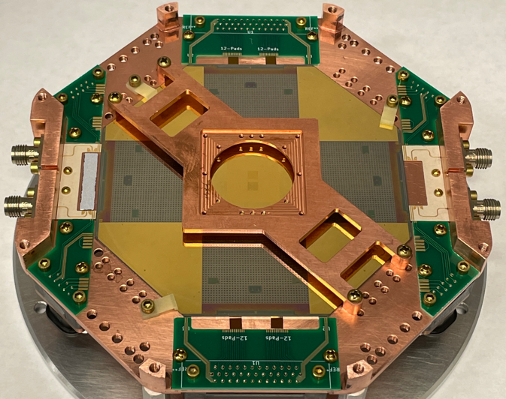
Very detailed information is now available from ultraviolet, optical, and submillimeter observations of the stellar, dust, and cold gas content of galaxies, and yet there is a dearth of understanding about the mechanisms that formed these galaxies. To truly understand how galaxies form, X-ray observations from high energy resolution imaging spectrometers are needed to see the cores of the galaxies themselves.
New large-area, high-angular-resolution, imaging X-ray spectrometers will expose the essential drivers of galaxy evolution, which leave imprints in the warm-to-hot plasma that cosmologists believe exists in the spaces between galaxies. These intergalactic spaces contain 40–50% of the 'normal matter' in the universe and extend well beyond the currently visible size of galaxies.
A class of X-ray spectrometers called microcalorimeters operate at a very low temperature—a few tens of milli-Kelvin above absolute zero. Over the past five years, the X-ray Microcalorimeter Group at NASA’s Goddard Space Flight Center (GSFC), the Advanced Imager Technology Group at Massachusetts Institute of Technology’s Lincoln Laboratory (MIT/LL), and the Quantum Sensors Group at the National Institute of Standards and Technology (NIST) in Boulder, Colorado have been collaborating on the development of an ambitious new X-ray camera with unprecedented imaging and spectroscopic capabilities.
This camera is based on a new type of X-ray microcalorimeter called a magnetic microcalorimeter. This NASA/MIT/NIST effort significantly extends the capabilities of the technology. For example, the X-Ray Imaging and Spectroscopy Mission (XRISM) mission, which is a collaboration between JAXA and NASA scheduled to launch in 2023, consists of a microcalorimeter array with 36 pixels. An ESA flagship mission currently in formulation (the Advanced Telescope for High Energy Astrophysics, or ATHENA) will have a microcalorimeter array with about two-thousand pixels. The arrays under development by the NASA/MIT/NIST collaboration have around one-hundred thousand pixels or more, reaching the angular scales and array sizes normally only associated with charged-coupled device (CCD) cameras.
Figure 2 shows one of the 100,000-pixel arrays the team has developed. The pixels are designed to have energy resolution that is about two orders of magnitude greater than that of an X-ray CCD camera. This exquisite high-energy resolution is critical to measure the abundances, temperatures, densities, and velocities of astrophysical plasmas. Such measurements will expose the essential drivers of galaxy evolution that are hidden in the plasmas of the universe.
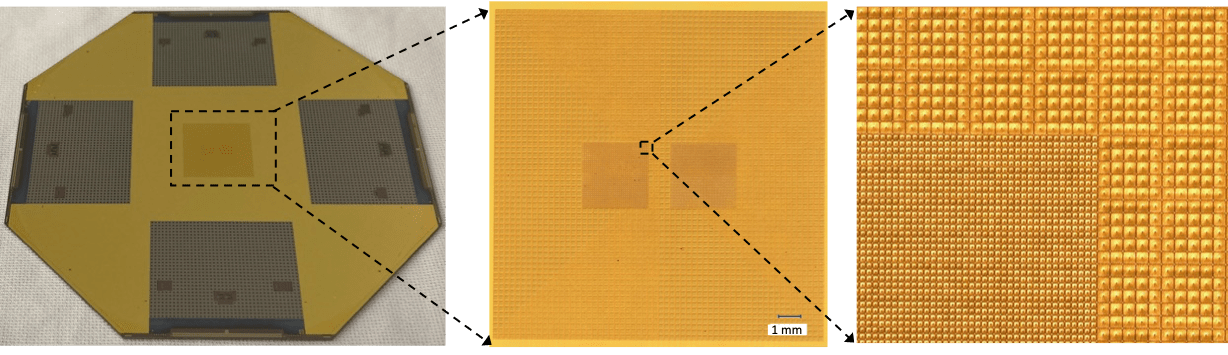
When an incoming X-ray hits the microcalorimeter’s absorber, its energy is converted into heat, which is measured by a thermometer. The temperature rise is directly proportional to the X-ray’s energy. The thermometers employed with magnetic microcalorimeters use paramagnetism to enable high-precision temperature sensing. In a paramagnet, the magnetization is inversely proportional to temperature, making it very sensitive to small changes at the low temperatures (at or below 50 milli-Kelvin) at which these devices operate.
In addition to single-pixel sensors, it is possible to design position-sensitive magnetic microcalorimeters in which a sensor is attached to multiple X-ray absorbers with different thermal conductance strengths. The unique temporal response of the different pixels to X-ray events allows the pixel event location to be distinguished. Due to the many heads in the sensor, this kind of thermal multiplexing device is often called a `Hydra,’ after the multi-headed serpentine water monster in Greek and Roman mythology. An example of a magnetic calorimeter `Hydra’ sensor is shown in Figure 3.
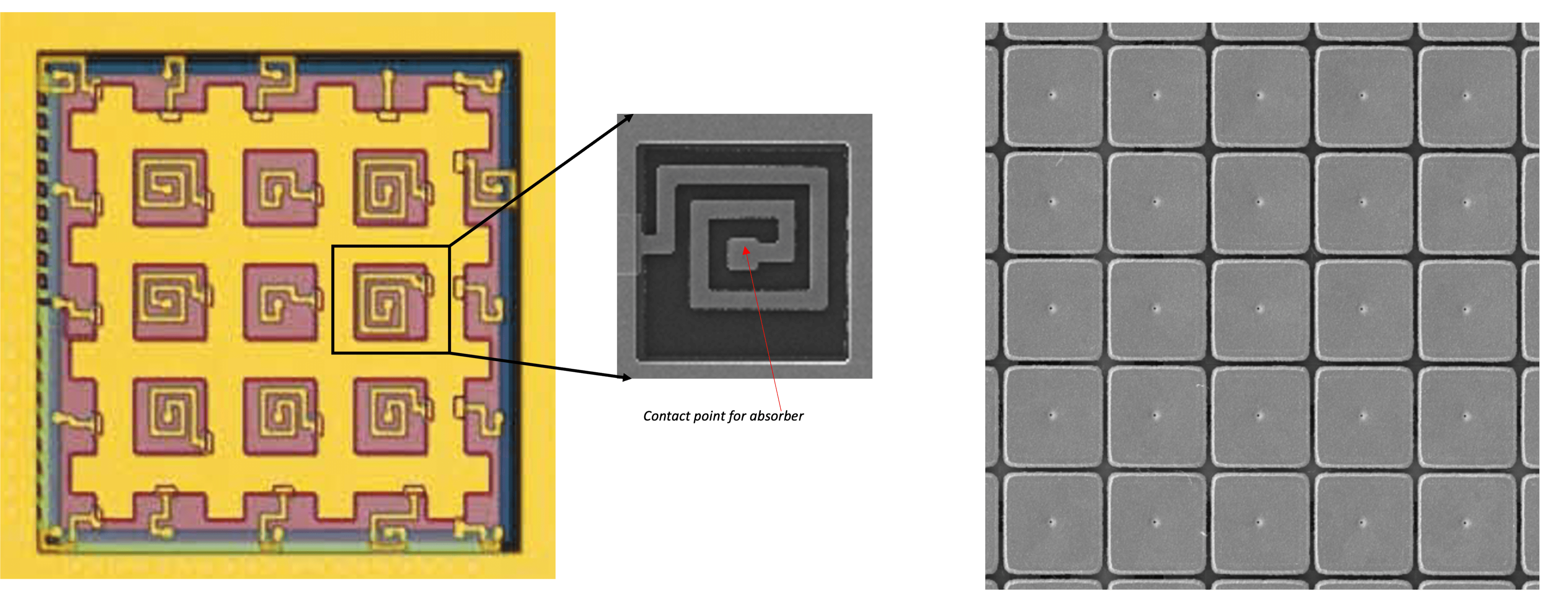
The main factors limiting the development of microcalorimeter arrays with the desired size and angular resolution (the distance from the center of one pixel to the next, or “pitch”) are the challenges involved in fabricating high-density, high-yield, microstrip superconducting wiring to connect all the pixels in the array. The major innovation employed to overcome this difficulty is to incorporate many layers of buried wiring underneath the top surface of detector chips on which the microcalorimeter arrays are then fabricated. Through an investment in technology for superconducting electronics, MIT/LL developed a process that allows over eight layers of superconducting wiring with high yield. The array shown in Figure 2 uses four layers of superconducting wiring, and the next generation of devices currently in fabrication utilizes seven layers of buried wiring. By combining this buried wiring process with the 25-pixel, ‘thermally multiplexed,’ microcalorimeters developed at NASA GSFC, the team has been able to produce large-format arrays of wires with pitch as fine as 25 microns.
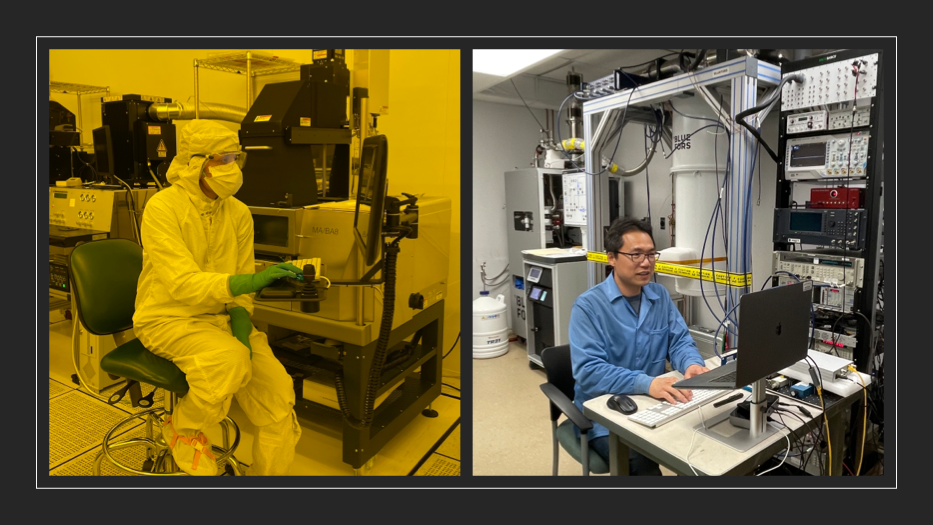
The final key development needed to make this detector suitable for future astrophysics missions is the multiplexed read-out needed for such large arrays of pixels. With NASA funding, NIST is developing a microwave-multiplexer superconducting quantum interference device (μ-MUX SQUID) read-out in a form-factor suitable for direct integration with this detector. Four two-dimensional chips carrying this read-out will be bump-bonded to the four large green rectangular areas in the outer regions of the detector shown in Figure 1. NIST has recently demonstrated low-noise μ-MUX SQUIDs, in small one-dimensional resonator arrays suitable for the new magnetic calorimeters. These μ-MUX SQUIDs measured magnetic flux noise that corresponds to just 20 quanta (or photons) at signal frequencies, meeting the challenging design requirements. In the near future, two-dimensional versions of this read-out will be bump-bonded to the detector shown in Figure 1, and the team hopes to demonstrate a ground-breaking new astrophysics instrumentation capability.
NASA’s Astrophysics Division has invested in magnetic calorimeter development at GSFC since 2008, most recently in this collaborative effort with MIT/LL. This type of device is under consideration for use in several potential future missions.
PROJECT LEADS:
Dr. Simon Bandler, Dr. Thomas Stevenson, Dr. Archana Devasia and Dr. Wonsik Yoon (GSFC), Dr. Kevin Ryu (MIT/LL), Dr. Joel Ullom, Dr. Benjamin Mates (NIST).
SPONSORING ORGANIZATIONS:
Astrophysics Division Internal Scientist Funding Model (ISFM) and Astrophysics Research and Analysis (APRA) Programs